- Author
- A.N. Other
- Subjects
- Ship design and development, Naval technology
- Tags
- None noted.
- RAN Ships
- None noted.
- Publication
- June 2019 edition of the Naval Historical Review (all rights reserved)
By Lieutenant M. De Angelis, RFD, RANR
Mario De Angelis enlisted in the Royal Australian Naval Reserve, Melbourne Port Division on 22 January 1974. Initially joining as a Cook, he later qualified as a Seaman Quartermaster Gunner and was commissioned as a Seaman Officer in 1976; he continues to serve as an Active Reservist.
Mario served in various ships including Patrol Boats, Landing Craft, Destroyers and the Aircraft Carrier HMAS Melbourne. In 1985, he changed to the Supply Branch with subsequent promotion to the rank of Lieutenant in 1987.
In recent times he has also used his civilian expertise in communications and logistics whilst serving in various short appointments including the Australian Warfare Centre at RAAF Williamtown, Support Command Australia and HQ Joint Logistics Command.
This essay is a brief review on the status of Autonomous Ships. We discuss the definition of the class, degrees of autonomy and the implications.
The lead countries and companies have high expectations of the economic returns from their investments in this field. To date, there are significant investments in both military and commercial fields.
Players in this industry need to consider a variety of technologies to resolve the risks and issues in communications, control and propulsion. These have a direct bearing on the levels of human involvement and the degree of automation.
The uptake of autonomous shipping has similarities with the uptake and development of autonomous land vehicles. The significant difference is that sea craft generally have a longer half-life than land-based vehicles. Technology take up is also slower. Interactions between autonomous vessels and their crewed counterparts will also affect the uptake timeline. Vehicle and vessel servicing also share similarities given the mix of technologies.
Each degree of automation up to complete autonomy requires increases in sophistication, data and redundancy. In the military context, damage control can mean the difference between mission success and mission failure.
The piracy risk is also increased. Not only are there pirates on the water to consider there are pirates in the data environment. From industrial sabotage to state sponsored hacking the new electronic environment offers a fresh hunting ground. With greater strategic reach, there is a proportional increase in risk.
The industry uses various scales to define automation and autonomy. The Technical University of Denmark (DTU) developed their scale for ship automation using the Society of Automotive Engineers (SAE) automation scale for land vehicles.
Table 1 illustrates the five-step classification of automation leading to full autonomy for land-based vehicles. DTU used this in their 2016 A Pre-analysis on Autonomous Ships. Funding from the Danish Maritime Authority helped publish the report. It describes how to define the various levels of autonomy and the potential of autonomous ships.
DTU developed a nine step series of classification terminology for merchant ships achieving full autonomy.
DTU used the terminology summarised in Lloyds Register 2016, to determine the classifications in Table 2. This resembles the SAE codes used for land vehicles.
Tables 1 and 2 show that greater levels in automation and autonomy require more complexity in the solution. There will be a close relationship between massive databases and the automation algorithms.
DTU details are below, including the address of their website.Technical University of Denmark: DTU Electro, Elektrovej 326 and DTU Management Engineering, Produktionstorvet 426, DK-2800. Kongens Lyngby www.dtu.dk
Commercial and Military Leaders
Commercial groups are primarily interested in the logistics side of cargo carrying autonomous vessels. Military elements are primarily interested in weapons delivery against military targets.
Label | Level of Automation | Description |
SAE 0 | No automation | The driver performs all driving and navigation without any aids. |
SAE 1 | Driver assistance | The car can keep a distance to other similar cars. |
SAE 2 | Partial automation | The car can perform simple tasks on its own, such as driving in the road system where it is located. |
SAE 3 | Conditional automation | The car can drive on its own in specific situation (e.g. on motorways). The driver does not need to control the car actively or to keep a lookout, but must be able to intervene at short notice. |
SAE 4 | High automation | The car can drive on its own in specific surroundings, but the driver does not need to be ready to take over control. |
SAE 5 | Full automation | Driverless cars in all surroundings and in all potential situations. |
Table 1: SAE scale of land vehicle levels of automation up to complete autonomy
Code | Description | Operator Role |
AL 0 | Manual steering. Steering controls or set points for course, etc. are operated manually. | The operator is on board or performs remote control via radio link. |
AL 1 | Decision-support on board. Automatic steering of course and speed in accordance with the references and route plan given. The course and speed are measured by sensors on board. | The operator inserts the route in the form of “waypoints” and the desired speed. The operator monitors and changes the course and speed, if necessary. |
AL 2 | On-board or shore-based decision support.
Steering of route through a sequence of desired positions. The route is calculated so as to observe a wanted plan. An external system is capable of uploading a new route plan. |
Monitoring operation and surroundings. Changing course and speed if a situation necessitates this. Proposals for interventions can be given by algorithms. |
AL 3 | Execution with human being who monitors and approves. Navigation decisions are proposed by the system based on sensor information from the vessel and its surroundings. | Monitoring the system’s function and approving actions before they are executed. |
AL 4 | Execution with human being who monitors and can intervene. Decisions on navigation and operational actions are calculated by the system which executes what has been calculated according to the operator’s approval. | An operator monitors the system’s functioning and intervenes if considered necessary. Monitoring can be shore-based. |
AL 5 | Monitored autonomy. Overall decisions on navigation and operation are calculated by the system. The consequences and risks are countered insofar as possible. Sensors detect relevant elements in the surroundings and the system interprets the situation. The system calculates its own actions and performs these. The operator is contacted in case of uncertainty about the interpretation of the situation. | The system executes the actions calculated by itself. The operator is contacted unless the system is very certain of its interpretation of the surroundings and of its own condition and of the thus calculated actions. Overall goals have been determined by an operator. Monitoring may be shore-based. |
AL 6 | Full autonomy. Overall decisions on navigation and operation are calculated by the system. Consequences and risks are calculated. The system acts based on its analyses and calculations of its own capability and the surroundings’ reaction. Knowledge about the surroundings and previous and typical events are included at a “machine intelligent” level. | The system makes its own decisions and decides on its own actions. Calculations of own capability and prediction of surrounding traffic’s expected reaction. The operator is involved in decisions if the system is uncertain. Overall goals may have been established by the system. Shore-based monitoring. |
Table 2: Autonomy levels (AL) extract from DTU ‘A Pre-analysis on Autonomous ships’.
The major commercial entities investing in autonomous ships are Kongsberg Marine (Norway), Rolls-Royce (UK), MUNIN a European based consortium, a UK consortium comprising the University of Southampton, QinetiQ and Lloyd’s Register.
National entities include the Danish Maritime Authority, the US Navy, China, the United Kingdom, Norway, Japan and the European Community. There is a mix of driving influences as all nations have both economic and military interests.
The following quote from the Port Technology website, (Maritime Information Services Ltd) demonstrates the level of state and commercial interest in autonomous vessels.
This week, Bloomberg reported that Japan’s largest container shipping company, Nippon Yusen Kabushiki Kaisha (NYK Line), is planning to test a remote-controlled vessel across the Pacific Ocean in 2019.
The Monohakobi Technology Institute, NYK Line’s research and development unit, is reportedly considering using a large container ship for the test from Japan to North America.
Interest is widespread and as diverse as the countries investing in the technology. Commercial interests centre on cost savings and increased profitability. Military interests centre on weapons delivery and interdiction of enemy sea assets, all with minimal risk to trained personnel.
Anything short of full autonomy is, in reality, a drone. This is similar to the USAF drones currently flying military missions. The pilot sits in a control centre and the drone transmits data from its sensor arrays to provide the pilot’s cockpit view.
A drone vessel would relay data from its sensor array to the shipping control centre to provide the bridge crew with the watch keeper’s view. This applies equally to the commercial or military application.
The main investment effort in drone and autonomous shipping is in the northern hemisphere. The main players are in the United States, Europe, Russia, Japan and China. The American, Japanese and European entities are in the public domain. Very little information is available from the Sino-Soviet sphere.
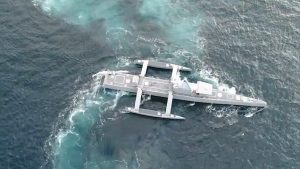
Autonomous communications, control and propulsion
Military applications for autonomous vessels require real time communication and control. Real time communication and control for merchant marine autonomous vessels is critical at points of departure and arrival. Long passages would mean quiet time with little or no change for a control centre to deal with. Greater autonomy requires more computing power. The minimum requirements are:
- Maritime Rules of the Road
- charts for all the oceans a vessel can expect to travel
- port information
- weather
- winds
- tides
This data needs correlation with the ship’s sensor array to confirm its status:
- fuel
- cargo condition
- sensor performance
- power availability
- speed through the water
- position by GPS, radar and sonar
- winds and tides experienced
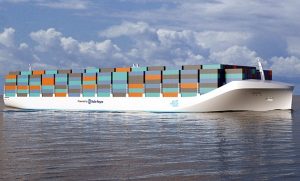
The size and mission of the autonomous vessel determine if data can be stored centrally ashore with the ship feeding information as it moves through an area or fully downloaded before departure. Coastal freighters need different data to that needed by a trans-Atlantic or trans-Pacific vessel. At departure and arrival points however, their data needs will coincide.
Propulsion options have a bearing on design and effort to maintain vessels operating efficiently. Fossil fuels require mechanical transfer of energy and management of toxic wastes. Electronic control and propulsion minimises energy loss through mechanical transfers but in a marine environment can be susceptible to electrical failure. Biofuels have the same issues as fossil fuels. Hydrogen fuel can operate in both mechanical and electronic environments.
Fossil fuels require tanks close to the engines and present an explosive or incendiary risk. Failures have catastrophic results.
Electronic propulsion relies on efficient battery power, and military diesel-electric submarines already use this technology. The Royal Australian Navy’s new Landing Helicopter Dock ships use electrical direct drive pods that remove the need for rudders. These have also demonstrated the critical risk of electronic failures during sea trials. Commercial shipping interests will baulk at risking valuable cargoes in this way. Reliability is a key economic consideration.
Biofuels, unlike fossil fuels, are renewable to the extent that production diverts crop growth from food to fuel production. Transport, storage and calorific values are much the same, and the same risks apply.
Hydrogen has more than twice the calorific value of carbon-based fossil fuels. NASA used it to propelthe Saturn 5 Apollo missions to the Moon. The tanks were huge and the hydrogen was under high pressure. At sea, this kind of storage is counterproductive as it displaces valuable cargo. The viable answer is a storage system at normal temperature and pressure utilising an electrical signal to release the fuel.
This works equally well for gas turbines, internal combustion or fuel cell technology. The tanks use metal-hydrides to soak up the hydrogen in a chemical lattice, much as a sponge absorbs water. These tanks hold more hydrogen by volume than an equivalent volume with hydrogen in gas or liquid form.
Ships on passage could refuel themselves in the same way that solar cells recharge batteries. Solar power could be used to trickle charge metal hydride tanks with hydrogen from the water resulting from its own combustion.
Economic arguments for autonomous ships
The economic argument is an offset of investment in technology against removal of human involvement in shipping. Autonomy simplifies ship design by removing the need for life support. No accommodation, food storage, bio-waste management, passages or environmental management are required. This leaves more ‘space’ for propulsion and cargo, increasing profitability by volume. Ships’ sea endurance is longer and they can traverse inhospitable environments, all without the cost burden of heating, cooling and life maintenance for humans.
In battle, autonomous ships can operate individually or as a pack to interdict or overwhelm enemy capability. Losing a few drones is politically easier to losing lives. Military tasking could also include hazard and explosives removal, hydrography and use in emergencies. Again, it comes down to cost effectiveness and risk compared with crewed ships currently performing those tasks.
Fuel type and propulsion systems are critical to the economic argument. These directly affect running costs and maintenance liabilities. Maintenance translates to human intervention. Autonomy seeks to eliminate this basic cost.
Autonomous capability, data and redundancy
Autonomous capability for a ship at sea translates to the manipulation of huge amounts of data. Ship handling characteristics are the starting point, along with the sensors and control systems to manage the ship. Automated radar and GPS readings require analysis and correlation with electronic copies of charts and positioning data. In addition, the shipping control centres require regular progress and situation reports, much as crewed ships do today.
Propulsion and data management need reliability and redundancy on a ship out of reach of human intervention. Mechanical or electronic systems need to perform at optimum levels. Equipment failures require self-diagnosis and repair or redundancy. Switching to the backup systems and business continuity is critical. This contributes to the economic argument.
In the event of complete failure, the autonomous vessel must have enough residual power to send a distress call to its home control centre. Equally, when help arrives, there must be enough power to authenticate access to the ships sensitive areas. Simple recognition of friend or foe.
Specific types of cargo fit into the calculations for power requirements. Sensitive cargoes that may spoil in the event of power failure need redundancy. Insurance companies will be very specific about cargo insurance where there is no repair or backup system in place.
Port facilities also need upgrading. Autonomous ships need to understand anchorage points and wharf designations. The autonomous ships will need security access by stevedores to both load and unload the various cargoes. Refuelling and other port services also require secure access.
Risks with autonomous ships
Risk exists for autonomous ships in the same way that it does for crewed ships. One exception is the risk posed by and to a live in crew. Autonomous ships will not need life support systems so there are no risks to a crew. Other risks range from interactions with crewed vessels, with military vessels, other autonomous vessels, sea and virtual piracy.
Crewed vessels range from pleasure craft, sailing vessels and crewed fishing vessels. These craft may not have the level of sophistication inherent in an autonomous vessel. The first time an autonomous vessel collides or interferes with a crewed ship will cause many reactions. Litigation over such an event will certainly affect the take-up of autonomous technology at sea.
Military vessels routinely board crewed merchant vessels. How will the military manage the interrogation and verification of cargoes carried by an autonomous vessel? Electronic records and cargo codes would need upgrading to avoid unnecessary interference with an autonomous vessel. Interrogation Friend or Foe (IFF) and data transfer facilities may need development for all territorial navies through which an autonomous vessel passes.
Pirates have a long, illustrious and ignominious history in the oceans of the world. The romance has faded and most piracy today involves smuggling illicit cargoes, armed interception of luxury craft and in some areas, boarding and ransoming of bulk carriers who stray within range of their usually smaller craft.
Criminal and State sponsored cyber warfare poses the next piracy threat against autonomous ships. Opposition military assets must be neutralised and commercial assets are valuable. Temptation will find new ways of extending bad behaviour.
Imagine holding an entire fleet of carriers to ransom. Pirates could congregate them or keep them moving. Worse, they could redirect them to a State where their cargoes would disappear.
Conclusion
Technological developments will continue to progress the advent of autonomous ships. The economic equations make investment in this field inevitable.
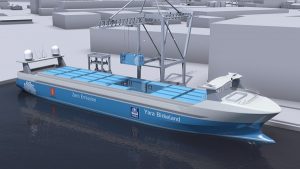
Land-based vehicles can have model turnarounds of as little as one year. Ships generally have a life span measured in decades. Some military forces refit their existing fleets to extend their service life. Autonomous vessels also follow this life cycle due to the investment they represent to their builders, owners and operators.
The risks to the take up and operation of autonomous ships will not stop their development. A combination of technologies with physical and software defences will keep the economic equation in favour of the market.
Military initiatives will probably involve both drones as well as autonomous fleet units. Autonomous ships will change the nature of convoys and revamp military manoeuvres as these new units come on line.
Autonomous ships will cause changes to Port facilities and maritime regulations. Their technological advances will also change the development of crewed vessels.